Quantum computers operate in a world where temperature is not just a dial on a thermostat, but a critical factor in performance and functionality. But why do quantum computers need to be cold? At their core, quantum computers rely on qubits that must exist in a stable, quantum state to perform calculations beyond the reach of classical computers. Any external ‘noise’, especially thermal noise, can disrupt these delicate states. By operating at extremely low temperatures close to absolute zero, these systems can preserve the fragile superposition of qubits and protect against environmental interference, ensuring they process information correctly. In this article, we’ll explore the fascinating reasons behind these icy operational needs and how they underpin the potential of quantum computing.
- Key Takeaways
- The Necessity of Sub-Zero for Quantum Processors
- Quantum Mechanics at a Glance
- Cooling Technologies in Quantum Systems
- Cryogenic Testing: Ensuring Quantum Readiness
- Alternative Approaches to Quantum Computing
- The Intersection of Quantum Computers and Classical Technology
- Summary
- Read More For More Information
- Frequently Asked Questions
Key Takeaways
Quantum computers require extreme cold to minimize thermal noise and environmental interactions that disrupt qubit stability, which is essential for maintaining superposition and quantum coherence.
Advanced cooling technologies like adiabatic demagnetization refrigerators and dilution refrigerators are critical for reaching the millikelvin temperatures necessary for quantum processors, and there is ongoing research to make these cooling methods more energy-efficient.
Alternative approaches and advancements in quantum computing, such as room-temperature qubits and hybrid quantum-classical systems, are being developed to improve performance, reduce stringent cooling needs, and complement traditional computing.
The Necessity of Sub-Zero for Quantum Processors


The necessity of sub-zero temperatures in quantum processors is not a design quirk but an essential requirement to maintain the stability and coherence of qubits. It all boils down to three primary factors: battling thermal noise, preserving the delicate state of superposition, and isolating the system from environmental interactions.
Thermal noise can disrupt the fragile quantum states of qubits due to particle vibrations at higher temperatures. To preserve the superposition state of qubits, the quantum system needs to be isolated from environmental interactions, such as heat and electromagnetic radiation, that can cause decoherence or loss of quantum information. Achieving ultra-low temperatures is paramount to minimizing the external influences that can disrupt qubit performance.
The Battle Against Thermal Noise
Imagine a class of students attempting to listen to a soft-spoken teacher amidst a cacophony of background noise. In this case, the students represent the qubits and the noise and thermal vibrations caused by heat. Just as the students would struggle to hear the teacher, the qubits find it challenging to maintain their quantum states amidst the disruptions caused by thermal noise.
When the temperature increases, particles in the quantum computer’s components experience more thermal vibrations. This can result in increased noise and errors in the quantum states of the qubits. Cryogenic temperatures, therefore, are not a choice but a necessity to ensure computational accuracy. This is why quantum computer components are tested at cryogenic temperatures to determine their thermal management capabilities, ensuring that any heat generated during operations does not affect performance.
Preserving the Delicate State of Superposition
The charm of quantum computing lies in qubits’ superposition property, allowing them to represent both 0 and 1 simultaneously. Quantum computers have a unique capability that allows them to process information in ways that classical computers cannot achieve. This gives them a distinct advantage in certain computational tasks. However, maintaining this delicate state of superposition is a daunting task, especially when faced with the heat from the environment.
Decoherence in qubits occurs when their quantum states are disrupted by heat and electromagnetic radiation, leading to a loss of quantum information. By maintaining extreme cold, quantum computers minimize these external influences, thereby preserving the superposition state of qubits. Moreover, low temperatures prolong the coherence time of qubits – the period during which they maintain their quantum properties – which is crucial for complex quantum computations.
Environmental Isolation for Quantum Coherence
Creating an environment conducive to quantum computing requires:
Orchestrating a symphony amidst a bustling city
Ensuring every little interaction with the environment doesn’t disrupt the delicate harmony of the quantum states, leading to decoherence.
Creating ultra-cold conditions to create an isolated environment for the quantum system
At low temperatures, quantum systems are less prone to interact with their environment, which could lead to decoherence. By achieving such ultra-low temperatures, we can preserve delicate quantum states and quantum coherence over longer timescales, thereby increasing the potential for successful quantum computation. Maintaining lower temperatures is, hence, critical to preserving quantum states and the future of quantum computing.
If want to learn about the possibilities of impacts that Quantum Computing & AI bring on our environment, please read our compelling article “Quantum Computing and AI Impacts & Possibilities“.
Quantum Mechanics at a Glance


Quantum mechanics is the foundation upon which the towering edifice of quantum computing is built. Two of its most fascinating phenomena – superposition and entanglement – are at the core of how quantum computers process information.
As mentioned earlier, superposition allows a quantum object to exist in multiple states simultaneously. This property is exploited in quantum computing, where qubits can simultaneously represent both 0 and 1. On the other hand, entanglement is a phenomenon where particles become interconnected, with the state of one impacting the state of the others. Quantum computing leverages this entanglement to link qubits and enhance computational probabilities.
Together, superposition and entanglement allow quantum computers to perform computations in a manner that could significantly outpace classical computers for certain problems.
Cooling Technologies in Quantum Systems


Achieving extremely low temperatures for quantum systems is no trivial task. It requires the application of specialized cooling technologies that can push the mercury down to temperatures as low as a few millikelvins – just a whisker above absolute zero.
The technology behind achieving such extreme cold involves the use of adiabatic demagnetization refrigerators and dilution refrigerators. These cooling systems are key to achieving the ultra-low temperatures needed for superconducting qubits in superconducting quantum computers.
Advanced models and simulations are also used to better understand heat and energy flow in quantum devices, enabling improved control and preservation of quantum processors.
From Dilution Refrigerators to Closed-cycle Cryostats
Dilution refrigerators and cryostats are the unsung heroes of quantum computing, often overshadowed by the more glamorous aspects of qubits and quantum algorithms. Yet, without them, quantum computing as we know it would be impossible.
Dilution refrigerators, such as the JDRY series, are employed to achieve the ultra-low temperatures needed by superconducting qubits in quantum computers through cryogenic cooling. They cool the system to within a few millikelvins of absolute zero, a feat that would make even the coldest regions of space seem balmy in comparison.
On the other hand, Cryostats play a crucial role in ensuring that components used in quantum computing can operate at temperatures close to absolute zero, a necessity for cryogenic testing and quality assurance.
The Quest for Energy Efficiency
While the focus has often been on achieving sub-zero temperatures for quantum computing, energy efficiency is another equally crucial aspect. After all, cooling to such low temperatures requires significant energy, and the quest for more efficient cooling solutions is an ongoing pursuit.
Cryogenics companies like Kiutra are at the forefront of this pursuit, developing scalable and sustainable cooling solutions for quantum technologies. Their offerings, such as the S-Type Optical cryostat, provide sub-Kelvin continuous cooling capabilities without relying on helium-3. The goal is to replace traditional supercomputers that consume megawatts of power with quantum computing systems that use only about 10 kilowatts, thereby making quantum computing powerful and energy-efficient.
Cryogenic Testing: Ensuring Quantum Readiness
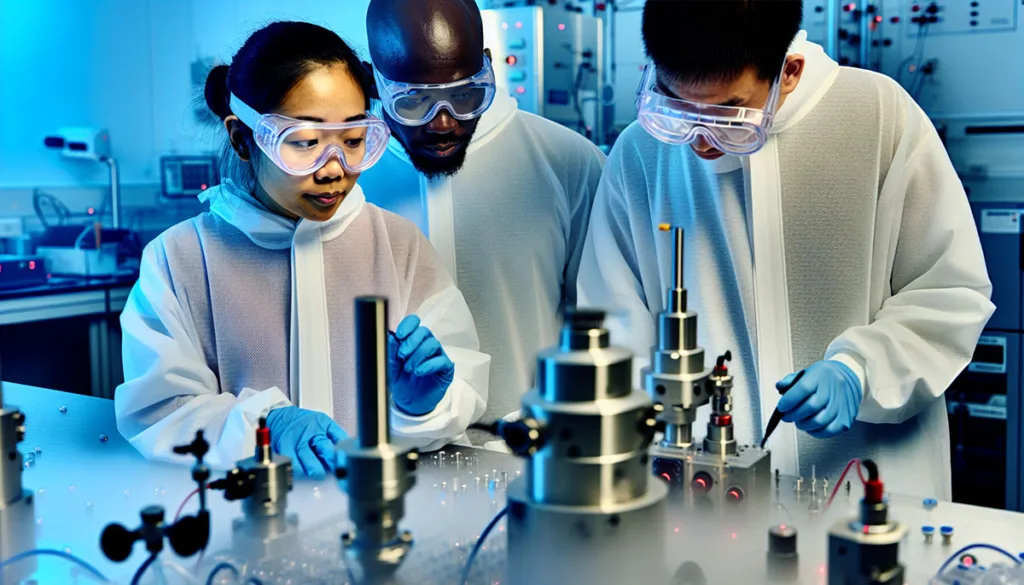
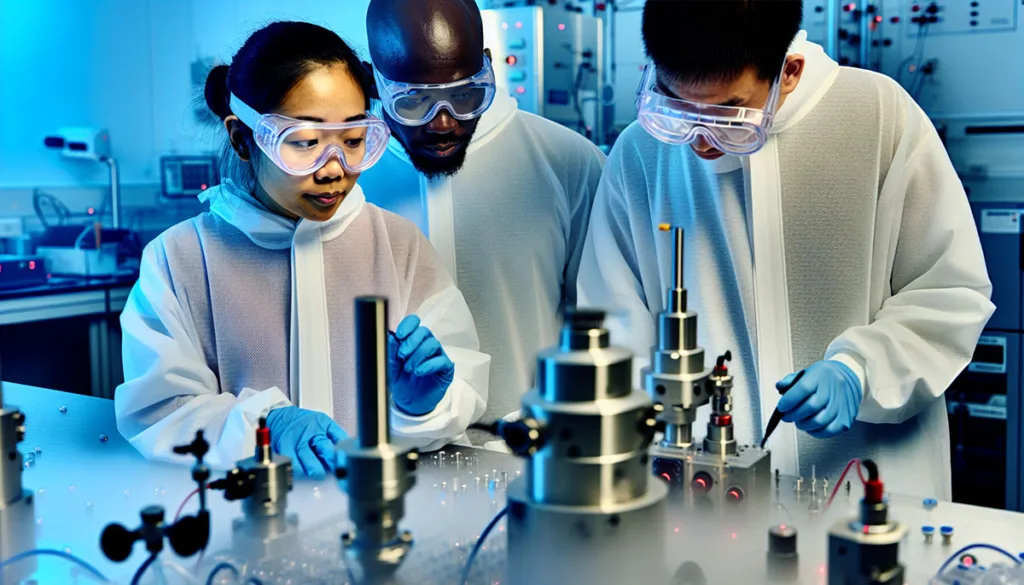
Before a quantum computer can solve complex problems, it must first overcome a challenge of its own: cryogenic testing. This process is a critical step in developing and practically applying quantum computing technology, ensuring the reliability and performance of quantum devices at ultra-low operational temperatures.
Cryogenic testing involves assessing the performance of components such as amplifiers and interconnects at cryogenic temperatures. This helps to verify key parameters like signal amplification, signal loss, and electrical conductivity, which are crucial for the quality of quantum hardware. Without such rigorous testing, the reliability and performance of a quantum computer would be uncertain, making cryogenic testing an integral part of the quantum computing journey.
Performance Under Pressure
Cryogenic testing is like a final exam for quantum computer components, testing their performance under the extreme cold conditions in which they are expected to operate. High-performance cryogenic probe stations, such as the HPD IQ3000 and Kilimanjaro 125, are the examination rooms for these components.
These cryogenic probe stations can support a range of applications relevant to quantum computing, including IR-sensor tests and radiometric tests. In essence, they assess whether the quantum components can perform effectively under pressure or, in this case, extreme cold.
The Quality Assurance Process
A comprehensive quality assurance process is paramount to ensure the reliability and performance of quantum devices. This process involves rigorous cryogenic testing, which provides critical data on coherence times, error rates, and fidelity, essential for designing efficient and reliable quantum hardware.
The quality assurance process helps to identify and address potential defects and temperature-related issues, ensuring that quantum devices function reliably once deployed in real-world scenarios. It is also crucial for reducing electronic noise that can interfere with qubit manipulation. In essence, cryogenic testing is a vital quality assurance measure that ensures the smooth operation of quantum computers.
Alternative Approaches to Quantum Computing
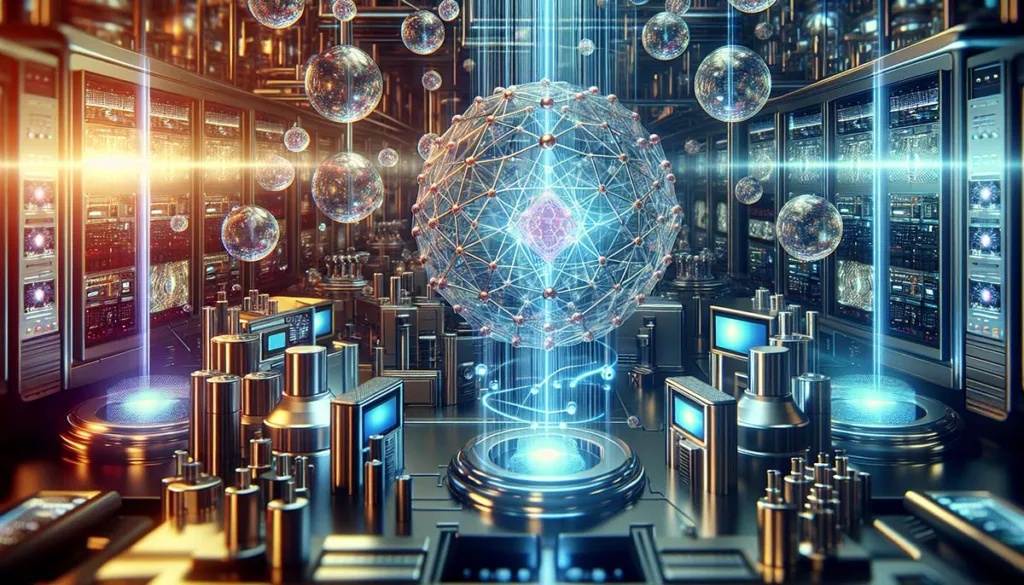
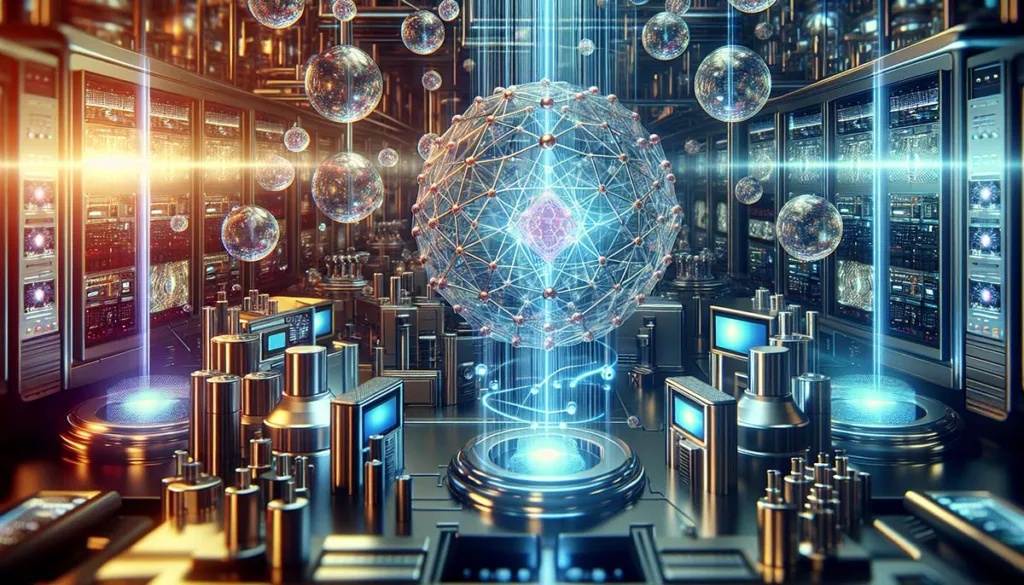
As revolutionary as traditional quantum computing may be, it’s not the only game in town. There are alternative approaches to quantum computing that aim to improve computational power and efficiency, each with its unique potential and challenges.
These alternative approaches offer exciting possibilities, such as topological quantum computing, adiabatic quantum computing, and quantum dot cellular automata. Instead of relying on superposition and entanglement, they leverage other quantum mechanical properties to perform computations, potentially allowing for better fault tolerance and less stringent temperature constraints.
Room Temperature Quantum Computing: A Future Possibility?
Imagine a quantum computer operating at room temperature. Sounds like science fiction, doesn’t it? Surprisingly, it could be a reality in the not-so-distant future. While the majority of quantum computers today operate at ultra-low temperatures, research is ongoing to develop quantum computers that can function at room temperature.
Developments in quantum computing are paving the way for exciting advancements in quantum technology. Some of the recent breakthroughs include photonic quantum computing and:
Molecular qubits: A metal-organic framework utilizing pentacene molecules and zirconium ions has achieved room-temperature quantum coherence in molecular qubits for over 100 nanoseconds.
Photonic qubits: Researchers are exploring using photons as qubits, which can be manipulated and transmitted over long distances.
Diamond-defect quantum computers: Diamonds with nitrogen-vacancy centres are being used as qubits, offering long coherence times and the potential for scalable quantum computing.
While these advancements are still in the early stages, they represent promising steps towards the development of more powerful quantum computers and keeping quantum computers functional for future applicants.
Breakthroughs in High-Temperature Qubits
While most qubits operate at ultra-low temperatures, a class of qubits can function at somewhat elevated temperatures: high-temperature qubits. These qubits, such as silicon spin qubits, offer potential advantages in quantum computing by reducing the cooling requirements.
Companies like Quantum Brilliance and XeedQ are already marketing desktop-sized diamond-defect quantum computers that can operate at room temperature, albeit with only a few qubits. Efforts are underway to increase this to hundreds of qubits in the future, promising exciting advancements in the field of quantum computing.
The Intersection of Quantum Computers and Classical Technology
Quantum computers are not meant to replace classical computers but to complement them. By performing complex calculations that are infeasible for classical computers, quantum computers augment the capabilities of their classical counterparts. Conversely, classical systems manage tasks like data handling and error correction, which are currently beyond the scope of quantum systems.
Integrating Quantum Processing Units (QPUs) with classical computing systems mirrors the historical strategy of deploying GPUs alongside CPUs to improve performance in high-performance computing. Organizations’ choice between implementing on-premises quantum computing or utilizing cloud-based quantum services will focus on meeting computational needs and security requirements within an integrated computing environment.
Bridging the Gap: Hybrid Systems
Just as a symphony orchestra combines a variety of instruments to create a harmonious whole, hybrid quantum-classical computing systems leverage the strengths of both to solve complex problems. Hybrid systems can optimise performance by assigning different aspects of a problem to the quantum and classical components.
Effective interaction between the quantum and classical components within hybrid systems is crucial and requires identifying specific control points in the algorithm. For instance, the Variational Quantum Eigensolver (VQE) is a hybrid algorithm that alternates tasks between quantum and classical computers, optimizing performance in fields like:
Chemistry
Material science
Optimization problems
Machine learning
By leveraging the strengths of both quantum and classical computing, hybrid algorithms like VQE have the potential to solve complex problems more efficiently.
The Role of Quantum Computers in the Current Tech Landscape
Quantum computing is already making inroads into various fields, including:
Material science
Financial modeling
Machine learning
Natural language processing
Logistics & manufacturing optimization
These applications are just the tip of the iceberg, with many more possibilities still in the exploratory stages.
In the financial sector, quantum computing is being explored for derivatives calculation and credit risk assessment. In machine learning, it is being used to accelerate algorithms. Natural language processing enables more efficient communication tools, and logistics optimizes tasks such as improved cargo loading processes. As the field of quantum computing continues to evolve, we can expect its impact on various sectors to grow correspondingly.
Summary
Quantum computing represents a paradigm shift in the field of technology, promising to redefine the future. However, its unique requirements, particularly the need for ultra-cold temperatures, present significant challenges. From battling thermal noise to preserving the delicate state of superposition, maintaining ultra-low temperatures is crucial to the successful operation of quantum computers.
While traditional quantum computers require ultra-low temperatures, research is ongoing to develop quantum computers that can function at room temperature. Furthermore, advancements in high-temperature qubits and hybrid quantum-classical systems highlight the field’s diversity and adaptability. As we continue to unravel the mysteries of the quantum world and harness its potential, one thing is clear: the future of computing is quantum, and it’s cooler than ever.
Read More For More Information
A new way for quantum computing systems to keep their cool
A quantum leap in cooling atoms for better computers
The Status of Room-Temperature Quantum Computers
Quantum Computer Temperature: Do They Need to Be Cold?
How Would Room-Temperature Superconductors Change Quantum Computing?